Biomedical Materials
Current Projects:- Nanoarchitected Cardiovascular Stents for Control of In-Stent Restenosis
- Microarchitected Iron Lattices For Magnetic Particle Capture
- Design and Additive Manufacturing of Bio-Scaffolds
- Designing Neural Probes for Long Term Neural Recording in Large Brains
- Stereolithographic Synthesis of Hydroxyapatite Bone Scaffolds
Nanoarchitected Cardiovascular Stents for Control of In-Stent Restenosis
Personnel: Akash Dhawan (Ph.D. student in Medical Engineering)
Cardiovascular diseases are some of the most prevalent and deadly in the United States. Many of these diseases involve the narrowing of important blood vessels, limiting the delivery of oxygen and nutrients to vital organs. The first attempts to solve this problem involved reopening the blood vessel lumen using bare metal stents made of steel or chromium delivered via catheter balloon angioplasty. Ideally, the stent would integrate into the blood vessel walls as endothelial cells regenerate over the initial lesion. While this bare metal stent method solved the problem initially, many stents would fail as early as a year after placement due to in-stent restenosis, which describes any reason for a blood vessel lumen to re-occlude. The most common reason for restenosis is neointimal hyperplasia, where smooth muscle cells pathologically proliferate to invade the stent and re-occlude the lumen. The modern solution to in-stent restenosis is the drug eluting stent, which releases drugs over time that mitigate smooth muscle cell growth. Some drawbacks to drug elusion include limited vessel healing and increased risk of thrombosis.
We plan to investigate the relationship between the mechanical properties of the stent and the effect on the vessel endothelial and smooth muscle cells. With this understanding, we can develop a novel stent coating that can utilize nanoarchitecture to control for substrate stiffness and mitigate smooth muscle cell restenosis without the use of drugs.
This work is in collaboration with Dr.Cindy Huynh in the lab of Professor Tejal Desai at the University of California San Francisco.
Microarchitected Iron Lattices For Magnetic Particle Capture
Personnel: Sammy Shaker (Ph.D. student in Bioengineering)
Functionalized magnetic nanoparticles have various applications stemming from the multiple modalities through which said nanoparticles can act and be acted upon. One application of interest involves functionalizing magnetic nanoparticles with various agents for in vivo chemotherapy capture, as demonstrated by several recent journal articles. Retrieval of these nanoparticles is likely to occur through the deployment of a magnetic device into the vasculature; however, there is a dearth of architected magnetic materials which could provide improved particle capture by spanning most of the width of a large vessel whilst allowing acceptable flow dynamics. In this project, the hydrogel infusion additive manufacturing method pioneered by the Greer group is applied to the synthesis of microarchitected magnetic alloys for this capture application. Iron-based alloys are the primary target of these efforts, and the effects of synthetic parameters on the magnetic properties of the synthesized materials as well as the capture efficiency of the synthesized lattices are targets of active investigation.
This work is performed in collaboration with Prof. Steven Hetts of the University of California San Francisco and Prof. Vitaly Rayz of Purdue University.
Design and Additive Manufacturing of Bio-Scaffolds
Personnel: Weiting Deng (Ph.D. student in Medical Engineering)
Physical cues at the local microenvironment are fundamental for cellular functions but can be challenging to competently control. Proper scaffold design would help identify the mechanisms of cell-extracellular matrix interactions in 3-dimensional environments. A key unsolved challenge has been the lack of quantifiable mechanical cues that could guide the fabrication of physiologically relevant scaffolds with high architectural complexity and anisotropy. We designed and fabricated spinodal-decomposition-inspired (spinodoid) biomimetic topologies with predictable anisotropic elastic modulus. We revealed that the design space possesses highly tunable directional-dependent stiffness with accuracy between the model predictions and test results upwards of 99%. Comparing the designed spinodoid structure with the bone sample obtained from micro-CT, it presented outstanding topological resemblance and exceptional anisotropic elasticity affinity. We anticipate this AI-based framework to be a starting point for more sophisticated biomimetic architecture designs with fully controllable mechanical hints and physiologically pertinent topologies in three dimensions that path the way to systematical investigation on complex tissue interactions for biomedical applications
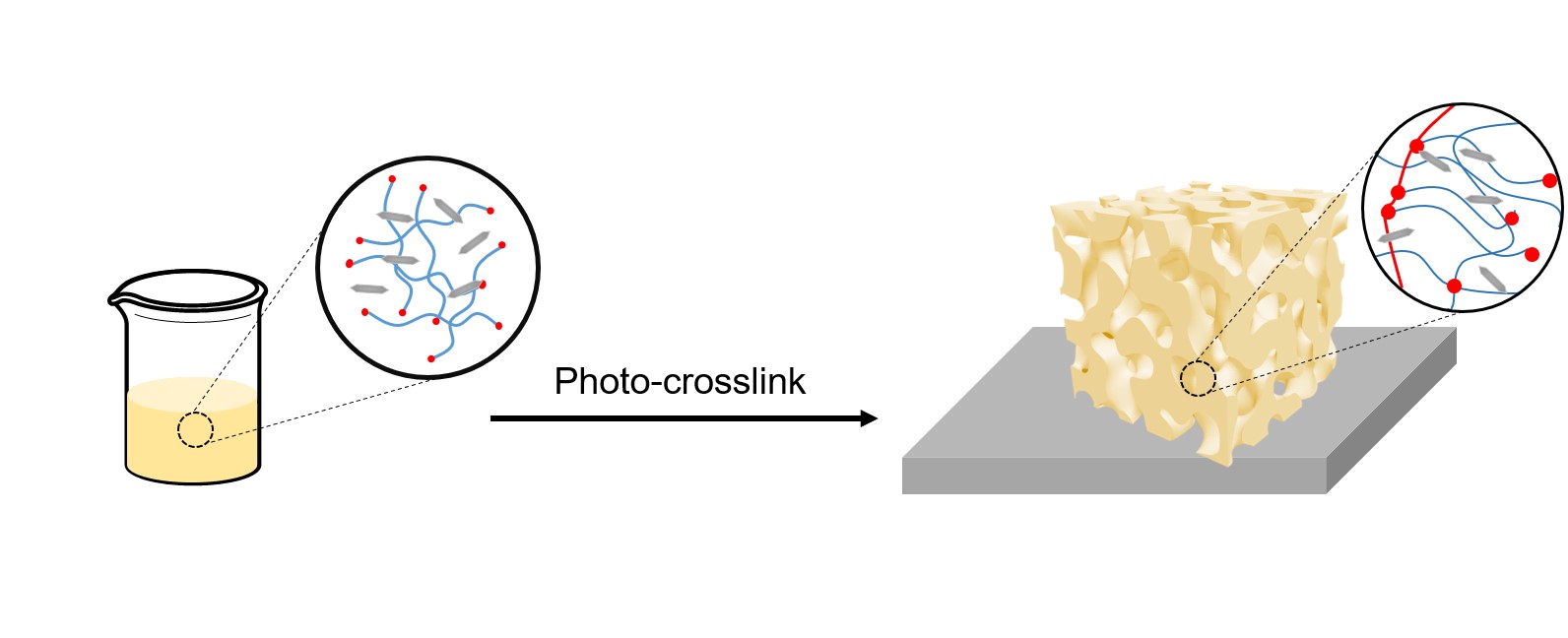