Mechanics and Physics of Nanomaterials
Projects:- Microstructure-Property Relationships in Additively Manufactured Nano-architected Oxides and Metals
- Emergent Nano-scale Phenomena and Engineering
- Characterization and Nanomechanical Properties of Unique Microstructures
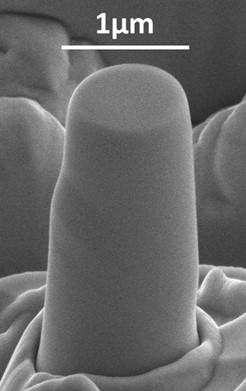
In the last decade or so it has been ubiquitously demonstrated that at the micro- and nano-scales, sample size dramatically affects material strength, as revealed by room-temperature uniaxial compression and tension experiments, as well as atomistic and continuum simulations, on a wide range of single-crystalline metallic nano-pillars. These studies provide a powerful foundation for understanding the fundamental deformation processes in materials with simple nanostructures (i.e. single crystalline metals); however, they are a far reach from representing real materials, whose microstructure is often complex, and contains boundaries and interfaces.
To tackle both these microstructural and nano-scale complexities, our research investigates the particular roles that the characteristic intrinsic material length scale and the external critical dimensions play on the mechanical response of materials through in-situ uniaxial compression and tension experiments performed at different temperatures on a variety of complex materials: from nanocrystalline ceramics to battery-relevant solids, shape memory materials, space-relevant materials, solid organics, and piezoelectric materials. We conduct nano-deformation experiments, either quasi-statically or dynamically, using SEM-based in-situ techniques and various custom-built apparatus to measure mechanically coupled properties at the micro- and nano-scale.
Current projects include a strong focus on additively manufactured materials and far-from-equilibrium microstructures:
- Microstructure-property Relationships in Additively Manufacture Nano-architected Oxides and Metals
- Emergent Nanoscale Phenomena and Engineering
- Characterization and Nanomechanical Properties of Far-from-Equilibrium Microstructures in Hydrogel-Derived Metals
Microstructure-Property Relationships in Additively Manufactured Nano-architected Oxides and Metals
Relevant Personnel: Rebecca Gallivan (Ph.D. candidate in Materials Science), and Wenxin Zhang (Ph.D. candidate in Mechanical Engineering)
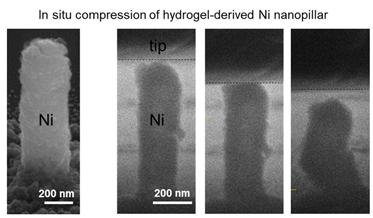
Recent developments from our group have enabled additive manufacturing of sub-micron resolution oxide and metallic materials, which offer high potential in areas of nanotechnology fabrication. These novel manufacturing techniques open new questions in how this unique class of processing influences the microstructure and properties of nano-architected materials. We have begun to explore the impact of this new set of methods on structure-property relations across a variety of materials including traditional FCC metals, metal oxides, and BCC metals.
Experiments focus on identifying yield and failure behavior as it relates to both extrinsic effect (structure size) and intrinsic effects (microstructural properties like porosity, grain size, etc.), characterizing specific microstructural features present in these nano-architected materials, and leveraging various simulation and modeling techniques to uncover mechanisms in nano-deformation.
Emergent Nano-scale Phenomena and Engineering
Relevant Personnel: Rebecca Gallivan (Ph.D. candidate in Materials Science)
At the nanoscale we see the emergence of unique behaviors due to increased relative importance of some mechanisms, a transition between discrete and continue behavior, and other size-related physics. Investigating these interplays is critical for both understanding and engineering novel and multifunctional material properties.
One example is our work in nanocrystalline nano-architected ZnO which shows an emergent electromechanically coupled behavior. Using both custom in-situ electrical data measurements and TEM-based microstructural investigation, we identify the key parameters behind this phenomenon and build a model for predicting the piezoelectric response of the structures. This work is one of several examples which indicate both the opportunity and need for deeper investigation of material properties in order to expand the materials selection for complex and multi-functional nanotechnology designs. These studies also focus on fundamental exploration to provide insight to the underlying mechanisms of material behavior and to lay the foundation for future design and engineering of these materials on the nanoscale.
Characterization and Nanomechanical Properties of Unique Microstructures
Relevant Personnel: Rebecca Gallivan (Ph.D. candidate in Materials Science), Thomas Tran (Ph.D. candidate in Materials Science), and Seneca Velling (Ph.D. student in Materials Science)
Current ongoing projects in this area are focused on characterizing far-from-equilibrium microstructural features in hydrogel-enabled additive manufactured metals and alloys. This works primarily focused on boundaries (twin, grain, phase, etc.) but addresses various microstructural features while investigating the relationship of processing and microstructure in these kinetically trapped materials. (image shows EBDS mapping of a highly twinned additively manufactured Cu sample)
Additionally we study the nanomechanical response of these materials through various ex-situ and in-situ techniques to both probe the fundamental role of boundaries and plasticity mechanisms and investigate the contributions of complex microstructures to engineered and enhanced material properties.